All cells on Earth possess a carbohydrate coat. Emerging evidence indicates that this coat serves as a critical conduit of information, but little is known at the molecular level. Elucidating how carbohydrates are assembled, how they are recognized, and how they function are issues at the scientific frontier. Using ideas and approaches that range from synthetic chemistry to cell biology, our research group is addressing the critical issues at this frontier.
Chemistry to Explore and Exploit Multivalent Protein–Carbohydrate Interactions
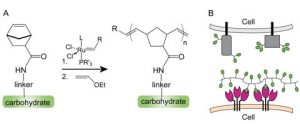
We reasoned polymer chemistry would be a powerful approach to assemble multivalent carbohydrate derivatives. We sought to devise a method to synthesize multivalent ligands with control over length and valency. Most polymerization reactions are incompatible with the polar functional groups of carbohydrates and give rise to mixtures of polymers that vary widely in valency and length. We surmised that the ring opening metathesis polymerization (ROMP) could be ideal for generated defined multivalent carbohydrate displays. ROMP can be a living polymerization, a reaction in which chain elongation occurs more readily than termination. As a result, we can use ROMP to assemble carbohydrate-substituted polymers with defined lengths, valencies, and arrangements of functional groups (Fig. 2). Our first indication of the power of ROMP for making bioactive polymers was our finding that ROMP can be used to generate carbohydrate-substituted polymers that block cell–cell interactions. Our subsequent studies underscore the versatility of this synthetic strategy for generating multivalent carbohydrate derivatives with tailored biological activities.
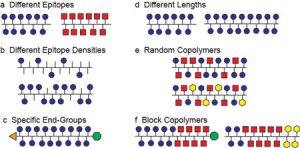
We used ROMP to create polymers that varied in chemical structure to probe the mechanisms underlying multivalent binding. Multivalent interactions had been thought to be non-selective, but our data challenge this paradigm. We found that multivalent ligands can by highly specific. Moreover, we used our synthetic expertise to carry out the first comparison of how the architecture of multivalent ligand influences its function. One finding to emerge from this investigation is that multivalent ligands generated by ROMP are especially effective at clustering proteins. We subsequently synthesized multivalent carbohydrate displays that inhibit a target carbohydrate-binding protein (L-selectin) by a novel mechanism: they cluster the carbohydrate-binding protein and induce it proteolytic release from the cell surface. This method of altering protein function had been unprecedented and it prompted us to consider using multivalent ligands to explore signaling pathways.
Multivalent Carbohydrates As New and Powerful Probes of Signal Transduction
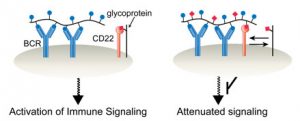
Such ligands, which can be used like small molecules for temporal control, can illuminate how protein assembly regulates cellular responses. For example, we used carbohydrate-based multivalent attractants to reveal how bacteria sense attractants and repellents. We found that a single receptor type is not sufficient to sense a specific attractant – an array of receptors is required for proper sensing and movement toward attractants. In effect, the clustered receptors act as a type of sensory organ (nose) that allow bacteria to respond sensitively and appropriately to stimuli in the environment. We have recently created multifunctional compounds that can co-cluster multiple copies of a carbohydrate-binding protein with copies of another signaling receptor (Fig 3). We synthesized compounds that promote the formation of specific multiprotein complexes to down-regulate signaling on immune cells. Thus, we can use our knowledge of synthetic chemistry and the cellular building blocks to assemble complexes that augment immune responses (for vaccine development) or attenuate them (for treatment of autoimmune diseases.)
Carbohydrate Polymer Biosynthesis: A New Target for Anti-Tuberculosis Agents
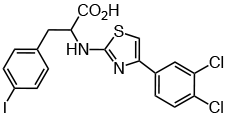
The resulting compounds block mycobacterial cell growth. Our investigations identify a new drug target for treatment of mycobacterial diseases, including tuberculosis.
Carbohydrate Polymer Biosynthesis: Controlling Length
Carbohydrate Polymers and Human Embryonic Stem Cell Pluripotency
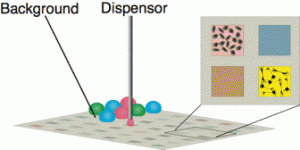
We used our arrays to identify surfaces that would support the growth of human embryonic stem (hES) cells. The ability to grow human cells in culture is the basis for remarkable advances in fields ranging from cell biology to medicine. Human ES cells are remarkable because they can be cultured indefinitely and can differentiate into every cell type in the body. The ability to propagate hES cells provides new opportunities to test drug candidates on human cells and to generate cells for therapies. Still, to exploit the full potential of hES cells for regenerative medicine, developmental biology, and drug discovery, chemically defined culture conditions are needed. Reproducible cellular responses depend on having a fully defined environment. In addition, contamination of animal cells and/or animal-derived components typically used for hES cell culture remains a safety concern. We have addressed the limitations of standard methods. Using our surface array strategy, we identified synthetic surfaces that can support the long-term culture of hES cells. A surprising result of this screen is the simplicity of the identified surfaces. They do not present complex mixtures of proteins, but rather display a single glycosaminoglycan-binding peptide. This finding was especially exciting to us because glycosaminoglycans are polysaccharides present on the surface of all mammalian cells. While others have examined surfaces that can interact with cell-surface proteins, our data indicate that carbohydrate polymers are important in pluripotency. The surfaces we devised highlight the critical role of cell surface polysaccharides in regulating cell fate decisions. They also offer a chemically defined environment for propagating hES cells. The simplicity and efficiency of our results led the Director of NIGMS to highlight them to Congress last year as an example of how basic science can yield economic and scientific benefits.